In our interdisciplinary and international research team of polymer chemists, physical chemists and materials scientists we are developing functional and intelligent polymer materials and devices.
One of the main aims is to control and manipulate structure-property relationships of hierarchical architectures from the molecular via the nanoscopic to the macroscopic device level.
Functionalities include:
- redox-activity for electrochemical sensors and electrocatalysis
- optical and electronic properties for polymer (opto)electronics
- stimuli-responsive behavior applicable as intelligent skin for controlled drug release in pharmaceutical applications and as actuators and sensors for soft robotics applications
Research Subjects
In addition to electropolymerization as versatile synthetic tool 1, we use in-situ and in-operando electrochemical analysis to study doping behavior of conducting polymer films.2 Cyclic voltammetry (CV) is combined with UV-vis-NIR spectroscopy (in-situ spectroelectrochemistry), in-situ conductance, quartz crystal microbalance (QCM) and spectroscopic ellipsometry measurements. AC-impedance and dc measurements further allow to decouple electronic and ionic conductivity in mixed conducting systems.3
We are for example interested in
- studying the optical signatures and energy levels, which is of key relevancy for organic photovoltaics 4 and nanophotonics.5
- the conductivity behavior and the tuning of conductivity by electrochemical doping.6
- the swelling behavior resulting from ion and solvent uptake during doping.2 This is particularly relevant for stimuli-responsive actuators and for studying electrochemistry in confinement.7
Electrochemical applications include actuators, sensors and (photo)electrocatalysis.
- J. Heinze et al. Chem. Rev. 2010, 110, 4724.
- C. Dingler et al. Macromolecules, 2022, https://doi.org/10.1021/acs.macromol.1c02515.
- M. Wieland et al. ACS Appl. Mater. Interf. 2020, 12, 6742.
- S. Albrecht et al. Adv. Mater. 2014, 26, 2533.
- J. Karst el al. Science 2021, 374, 612.
- D. Neusser et al. Chem. Mater. 2020, 32, 6003.
- J. Kuhlmann et al. Chem. Eur. J. 2021, 119, 163301.
Block copolymer films 1 with their ability to self-assemble into highly ordered microdomain structures on length scales between 5 and 50 nm are high performance mesoporous templates for electrodeposition of inorganic semiconductors such as TiO2, 2 bio-inspired material synthesis of functional oxides and (photo)electrocatalysis under confinement. Functional block copolymer templates include the incorporation of redox active blocks 3.
Additionally, we explore bio-inspired routes towards polymer/mineral hybrid materials by infiltration of block copolymer templates with inorganic species from aqueous solution at room temperature. Besides work on calcite we are interested in the synthesis of nanostructured functional oxides, 4 e.g. Co3O4 for electrocatalytic applications.5
Within the CRC1333 we are exploring the immobilization of redox-active species at the pore bottom and pore walls of block copolymer templates as well as of redox-active matrices by advanced electrochemical techniques.6
- S.S.Y. Lu, et al. Macromol. Rapid Comm. 2020, 41, 1900485.
- E.J.W. Crossland, et al. Nano Lett. 2009, 9, 2807. & Nano Lett. 9, 2009, 2813.
- E.J.W. Crossland et al. ACS Nano 2010, 4, 962.
- A. Finnemore et al. Adv. Mater. 2009, 21, 3928.
- A.S. Schenk et al. Nanoscale 2017, 9, 6334. & Cryst. Growth & Design 2020, 20, 10, 6407.
- L. Stein et al. Nanoporous Miktoarm Block Copolymer Templates with Redox-Active Matrix & Pore Wall Functionalization, Manuscript 2022 in prep.
In this research field we are developing and processing functional polymeric materials which can be triggered by external triggers, such as humidity 1, temperature and electric fields (incl. electrochemistry).2,3 This includes research on intelligent stimuli-responsive hydrogels, polyelectrolytes, thermoresponsive polymers, conducting polymers and mixed conducting materials.
On the one hand these materials shall be applied in intelligent devices for soft robotics applications. Bilayer devices were for example developed which change their curvature as function of the relative humidity.4 Here a intensive collaboration on the mechanical behavior is established with Prof. Steeb, Mechanics, University of Stuttgart. On the other hand controlled release of drugs is studied through articificial skin layers in close collaboration with Prof. Lunter, Pharmaceutical Technology, University of Tübingen.
- M. Wieland et al. ACS Appl. Mater. Interf. 2020, 12, 6742.
- C. Malacrida, et al. J. Mater. Chem. C 2020, 8, 15393.
- J: Karst et al. Science 2021, 374, 612. in collaboration with Prof. H. Giessen, Physics, USTUTT
- C: Dingler et al. Adv. Mater. 2021, Doi: https://doi.org/10.1002/adma.202007982.
Having a strong expertise in block copolymer self-assembly 1 we have focused on morphology control of semicrystalline polymers in recent years. To gain control over mesoscopic order in thin films of p-type polymers like p-type poly(3-hexylthiophene) and n-type P(NDI2OD-T2) aims at understanding structure formation starting from crystal structure analysis into macroscopic alignment.
Controlled solvent vapor annealing treatment 2 and crystallization under confinement 3 are some of the methods we use to reduce nucleation densities and align and order polymer films. Methods include atomic force microscopy (AFM), scanning electron microscopy (SEM) and X-ray techniques. The effect of chemical and electrochemical doping on the local morphology and anisotropy of charge transport are measured with field effect transistor (FET) and 4-point-probe conductivity techniques.
- S. Ludwigs et al. Nature Mater. 2003, 2, 744.
- E.J.W. Crossland, et al. Adv. Funct. Mater. 2011, 21, 518. & Adv. Mater. 2012, 24, 839.
- F.S.U. Fischer et al. Nanoscale 2012, 4, 2138.
- F.S.U. Fischer et al. Adv. Mater. 2015, 27, 1223
- Y. M. Gross, et al. Chem. Mater. 2019, 31, 3542.
- D. Neusser et al. Chem. Mater. 2020, 32, 6003.
Our fully equipped organic and polymer chemistry lab is specialized on chromophore synthesis and controlled polymerization techniques of functional (block) copolymers and semiconducting polymers. Tailor-made material design with architectural control is applied to further induce self-assembly and crystallization on hierarchical length scales in film geometries. Post-polymerization modification of polymers and films, e.g. by click chemistry, is among our specializations.
Research examples include:
- Triarylamine and carbazole redox polymers1
- N-type semiconducting polymers with varying regioregularity2
- Conjugated thiophene polymers with 2D- or 3D- architectures using oxidative, Ni-catalyzed or electro-polymerization methods3
- Conjugated polyelectrolytes4,5 for mixed conductivity
- Chromophores for electro-optical applications6
- C. Malacrida, et al. J. Mater. Chem. C 2020, 8, 15393.
- Y.M. Gross et al. Macromolecules 2017, 50, 5353.
- M. Scheuble et al. Macromol. Rapid Commun. 2015, 36, 115.
- T.V. Richter et al. J. Am. Chem. Soc. 2012, 134, 43.
- M. Wieland et al. ACS Appl. Mater. Interf. 2020, 12, 6742.
- C. Rothe et al. Phys. Chem. Chem. Phys. 2020, 22, 2283.
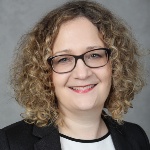
Sabine Ludwigs
Prof. Dr. rer. nat. habil.Executive Director of IPOC & Head of Chair